Best Paper Award at ECOS 2024
Highlights
Dareen and colleagues from Net-zero lab won the Best Paper Award in ECOS 2024 for the manuscript titled “Modelling The Effect of Future Uncertainty in Energy Prices on Decarbonization Pathways for Secondary Aluminium Production” by Dareen Dardor et al.
The awarded paper used several novel approaches to assess the effect of volatile energy markets on the selection and performance of decarbonization options in the aluminium industry. This work was developed as part of the NZLV consortium working in close collaboration with industry partners at Novelis Sierre.
IPESE researchers receiving the award: Dareen Dardor and co-author Daniel Florez-Orrego
Abstract
Aluminium is one of the most widely used metals in the world, but its production is highly energy intensive and largely dependent on the use of fossil fuels. A typical secondary aluminium production facility consumes 700 – 1,000 kWh of natural gas and 200 – 400 kWh of electricity per tonne of rolled aluminium sheets. Thus, in order to meet its global environmental targets, the aluminium industry is shifting towards alternative energy resources. Potential decarbonization routes include the use of biomass to replace fossil fuel via thermal gasification, the integration of carbon abatement and utilization units, the use of power-to-gas and energy storage systems, direct electrification of aluminium furnaces, installation of waste heat recovery units for power generation, among other alternatives. While most of these technologies have lifetimes of around 20 – 25 years, decisions on their installation must be made today. Biomass, electricity, and natural gas costs can be subject to unpredictable market variations, whereas carbon prices are related to both environmental regulations and future market situation. In this context, future uncertainty in energy prices should be accounted for in today’s decision-making. This work presents a systemic approach for assessing the effect of uncertain energy prices on the performance of different decarbonization routes for secondary aluminium production. To this end, a mixed integer linear programming (MILP) approach is used to generate a list of optimal system configurations under different economic conditions. Next, Monte-Carlo simulations are applied to predict representative price trends of commodities and to compute the resilience of each decarbonization scenario based on the likelihood of its occurrence under varying monetary circumstances. Results indicate that decarbonization pathways have a 50% probability to be cheaper than fossil-based CO2 emitting configurations. Moreover, the decarbonization option with the highest probability of being the economic best was the “Elec-bio” cluster which relies on a combination of electricity and biofuels to operate the plant’s furnaces. A probability of 22 – 37% was estimated for realizing a cheaper “Elec-bio” solution with respect to the natural gas driven base case over a lifetime of 25 years. Additionally, CO2 tax must not be the only economic incentive for emissions reduction but needs to be coupled with increased fossil fuel prices. Finally, this type of analysis allows decision makers to appreciate the potentials and risks associated with future decarbonization routes.
Key words: Aluminium, Decarbonization, Uncertainty, Energy prices, MILP optimization, Monte-Carlo simulation.
Overview
An extensive literature survey has been conducted to benchmark European market prices of the main utilities imported in our system, namely: natural gas, electricity, and biomass. The evaluation also included a review of CO2 tax based on the European Emissions Trading System (EU-ETS). The price of imposed CO2 tax directly influences the system’s total cost in the form of a penalty for continuing to emit GHGs. Figure 1 shows European benchmark prices for the relevant utilities excluding site specific tax and transportation fees. Predictions are blurred because these values are at best benchmarks. This is because the market is not only a function of demand and supply but is also dependent upon numerous geopolitical factors which are simply unpredictable in the long term. Nonetheless, prices are a major influencing factor on decision makers choices towards industry decarbonization which necessitates the development of a systematic methodology to deal with such uncertainty.
In this work, several decarbonization pathways have been assessed. A simplified schematic of the potential alternatives under evaluation is shown in Figure 2. This diagram is mainly focused on highlighting the interaction between the market and plant systems and doesn’t necessarily depict all interactions within the plant. Energy conversion technologies and furnaces have been modelled in detail using various available software such as Aspen plus® or Ansys Fluent. Industrial system audits and commercial technology specifications were also used as sources of information in building the optimization superstructure. Finally, a mathematical formulation of the system is defined using Lua programming language which is used in solving the MILP optimization problem. As depicted in Figure 2 the different alternatives considered within this system include: - Direct electrification: is a possible option for high temperature heating applications. Specific furnace solutions include electric radiant tubes for the holder, electric convective options for the pusher and ACL, and plasma for the melter furnace which is a lower technology readiness level (TRL) compared to the other solutions. - Power-to-gas solution: in this case proton exchange membrane (PEM) or alkaline electrolyzers using water and renewable electricity sources are employed to produce H2 gas consuming approximately 55 kWhElec/kgH2. The H2 can be burnt directly in H2 furnaces or combined with captured CO2 to produce synthetic natural gas (SNG). The SNG can be used directly or stored for seasonal demands based on the availability of excess electricity. - Biomass gasification systems: where woody biomass recourses are imported from the market and combusted or transformed into gaseous fuels through gasification in a dual-fluidized bed gasifier. Options include SNG, Syngas (a mixture of CO and H2), or H2. The former two are sent to either air-fuel or oxy-fuel furnaces while H2 is only burnt in air-fuel furnaces since no CO2 is present in the stacks. - Fossil based carbon capture scenarios: where natural gas from the grid is the main energy source while carbon capture and sequestration are used to mitigate the resulting emissions. Capture technologies modelled include conventional amine systems, membrane units, adsorption beds, or H2O condensation units following oxyfuel combustion furnaces. More information about these systems is given in (Dardor et al. 2024). - Any combination of the above systems: may constitute an optimal solution achieving decarbonization goals.
The capital investments of utilized plant units have been evaluated based on existing systems, published literature, and vendor information. These costs are included in the total cost optimization problem definition, for example a base price of 1,200 €/kWElec is assumed for the electrolyzer unit and around 1,300 €/kWbiom for the gasification system.
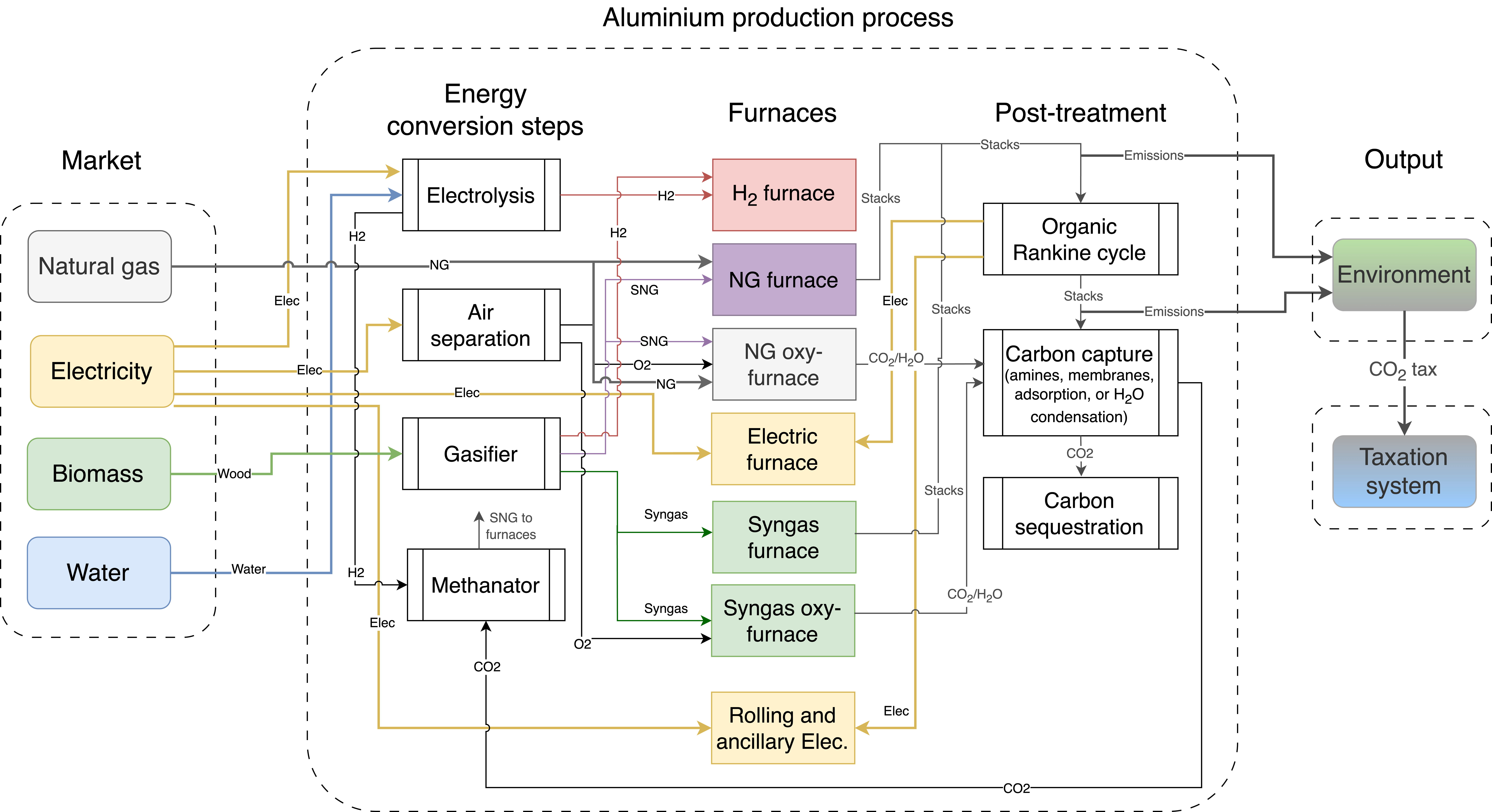
The effect of uncertainty in energy prices was modelled using two complementary approaches. First the MILP approach is used to inform us of the solution space and the probability of each solution being the best under the defined set of 4,000 price combinations. Moreover, we can locate ourselves using price maps that define the optimal solution configuration that minimizes total cost at each price level. Next, MCA is used to evaluate the future resilience of these solutions under stochastically changing prices over a lifetime of 25 years. Detailed findings and significance of these results are described below. A sample of the results obtained in the paper is depicted in Figure 3 describing the solution space where is was found that the Elec-Bio” configuration has a 58.3% probability of being the cheapest decarbonization option. This cluster mainly applies bio-syngas furnaces derived from biomass gasification and direct electrification furnaces. The second most common decarbonization solution (21.6%) is the “All-Elec” option that relies mainly on electricity utilization in a combined direct electrification and power-to-gas scenario. Further details of the utility price heat maps and resulting variations in system configuration are decribed in the full conference paper.
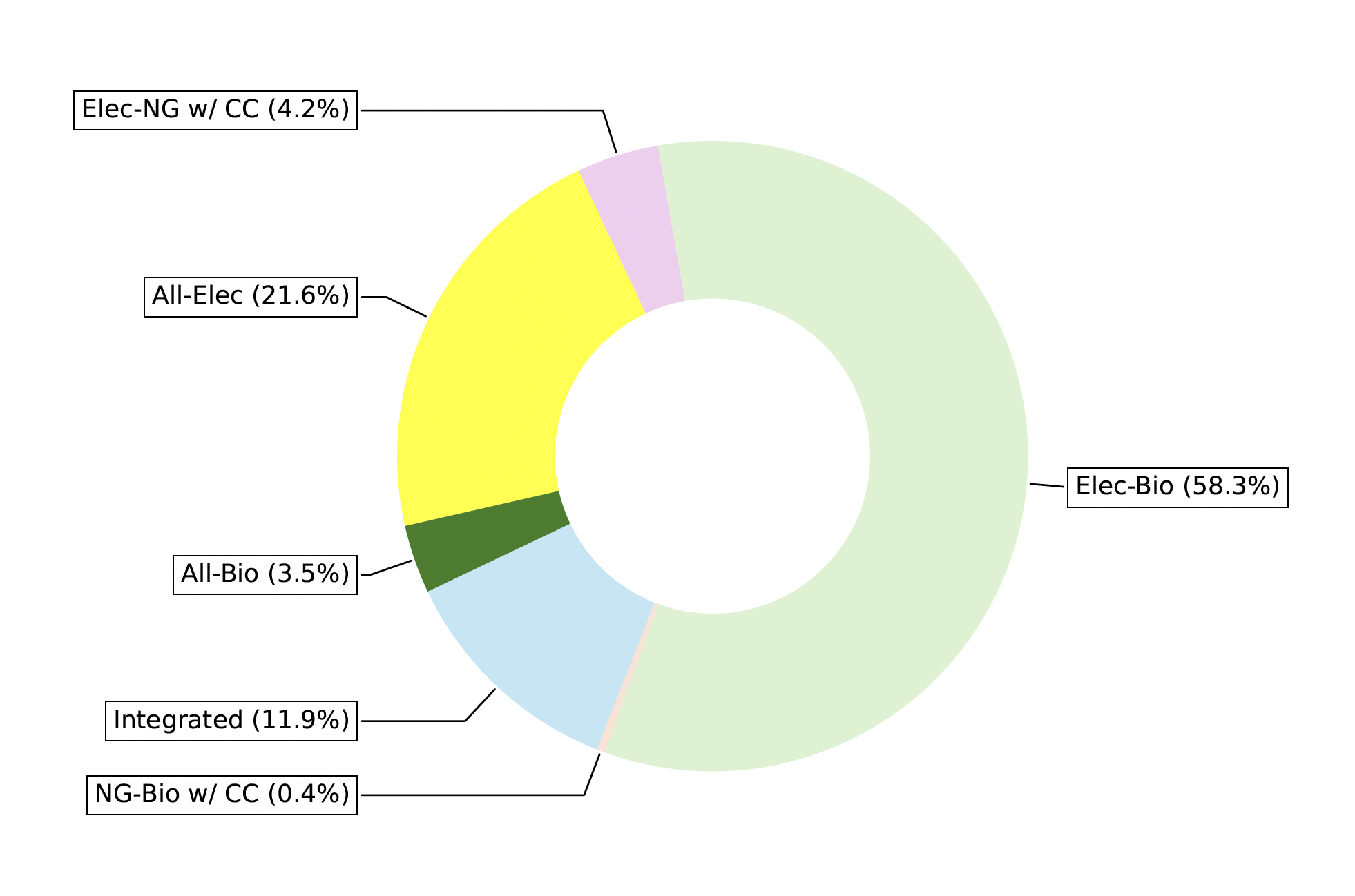
References
[1] Dardor D, Flórez-Orrego D, Aimone L, et al. Modelling The effect of future uncertainty in energy prices on decarbonization pathways for secondary aluminium production. In: 37th International Conference on Efficiency, Cost, Optimization, Simulation and Environmental Impact of Energy Systems. Rhodes, Greece, ResearchGate, 2024.
[2] Flórez-Orrego D, Dardor D, Germanier R, et al. A systemic study for enhanced waste heat recovery and renewable energy integration towards decarbonizing the aluminium industry. In: ECOS 2023 - The 36th international conference on Efficiency, Cost, Optimization, Simulation and environmental impact of energy systems. Las Palmas de Gran Canaria, https://infoscience.epfl.ch/record/303618?ln=en (2023).
[3] Dardor D, Flórez-Orrego D, Ribeiro Domingos ME, et al. Decarbonizing the production of primary aluminium using renewable resources. In: Proceedings of the 2023 AIChE Annual Meeting, American Institute of Chemical Engineering. Orlando (FL), United States, https://infoscience.epfl.ch/record/306686?ln=en&v=pdf (2023).
[4] Flórez-Orrego D, Dardor D, Ribeiro Domingos ME, et al. Renewable energy integration and waste heat recovery for the production of sustainable jet fuel and decarbonization of industrial heating applications. In: Proceedings of the 2023 AIChE Annual Meeting, American Institute of Chemical Engineering. Orlando (FL), United States, https://infoscience.epfl.ch/record/306479 (2023).
[5] Flórez-Orrego D, Dardor D, Ribeiro Domingos ME, et al. Renewable energy integration and waste heat Valorization in Aluminum Remelting Mills for the Co-Production of Kerosene and Methanol In: ESCAPE 2024 - 34th European Symposium on Computer Aided Process Engineering. Florence, Italy, https://infoscience.epfl.ch/record/311525 (2024)
[6] Dardor D, Flórez-Orrego D, Ribeiro Domingos, M, et al. CO2 Capture and management strategies for decarbonizing secondary aluminium production. In: ESCAPE 2024 - 34th European Symposium on Computer Aided Process Engineering. Florence, Italy, https://infoscience.epfl.ch/record/311522 (2024).
[7] Flórez-Orrego D, Dardor D, Aimone L, et al. Integration of renewable energy and reversible solid oxide cells towards decarbonizing the secondary aluminium production and urban systems. In: 37th International Conference on Efficiency, Cost, Optimization, Simulation and Environmental Impact of Energy Systems. Rhodes, Greece, 2024.